Our great luminary, the Sun powers its emissions by the fusion of hydrogen where the two hydrogen atoms combine, or fuse to become a helium atom. The fusion of the two hydrogen atoms into a helium atom releases energy. The hydrogen nucleus components of neutrons and protons combine to form the helium nucleus. The energy required to bind the two hydrogen atoms is more than the strong nuclear force needed to hold the helium atom together. Einstein applied the term ‘missing mass’ also called mass defect to describe the energy released when the new nucleus was formed.
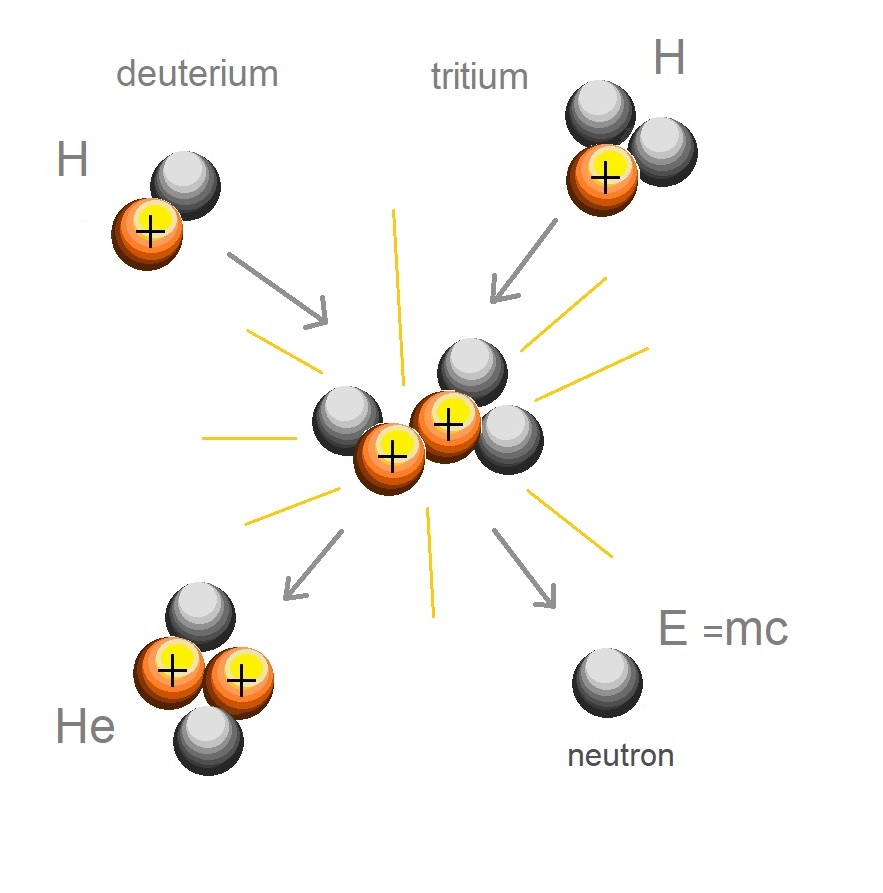
When hydrogen is burnt for power, i.e. combined with oxygen the product is water (unless it is burnt in air then nitrogen oxides are also produced). But coal and petrol produces carbon dioxide CO2, carbon monoxide CO, and a host of ‘nasties ‘ like; oxides of sulphur and nitrogen which dissolve in rain water and become acidic.
Carbon based fuels have been compressed in the Earth’s crust for millions of years, now in a hundred years we are releasing their burn by-products into our own air we breathe.
Photo synthesis the process plants use to power their conversion of water H2O and carbon dioxide CO2 into oxygen O2 and sugar CH2O is explained in detail in part3 Energy.
Ah but oil companies make a lot of money selling carbon based fuels to us. And the sun does not shine at night.
Hydrogen is the most common fuel source in our universe. Our sun uses 500 million metric tons each second. [1]
What are the products from burning hydrogen? Is it just water?
Do we have to change our engine technologies to burn hydrogen? Yes our atmosphere is 79% nitrogen.
Who processes hydrogen for sale? These are questions that come to my mind.
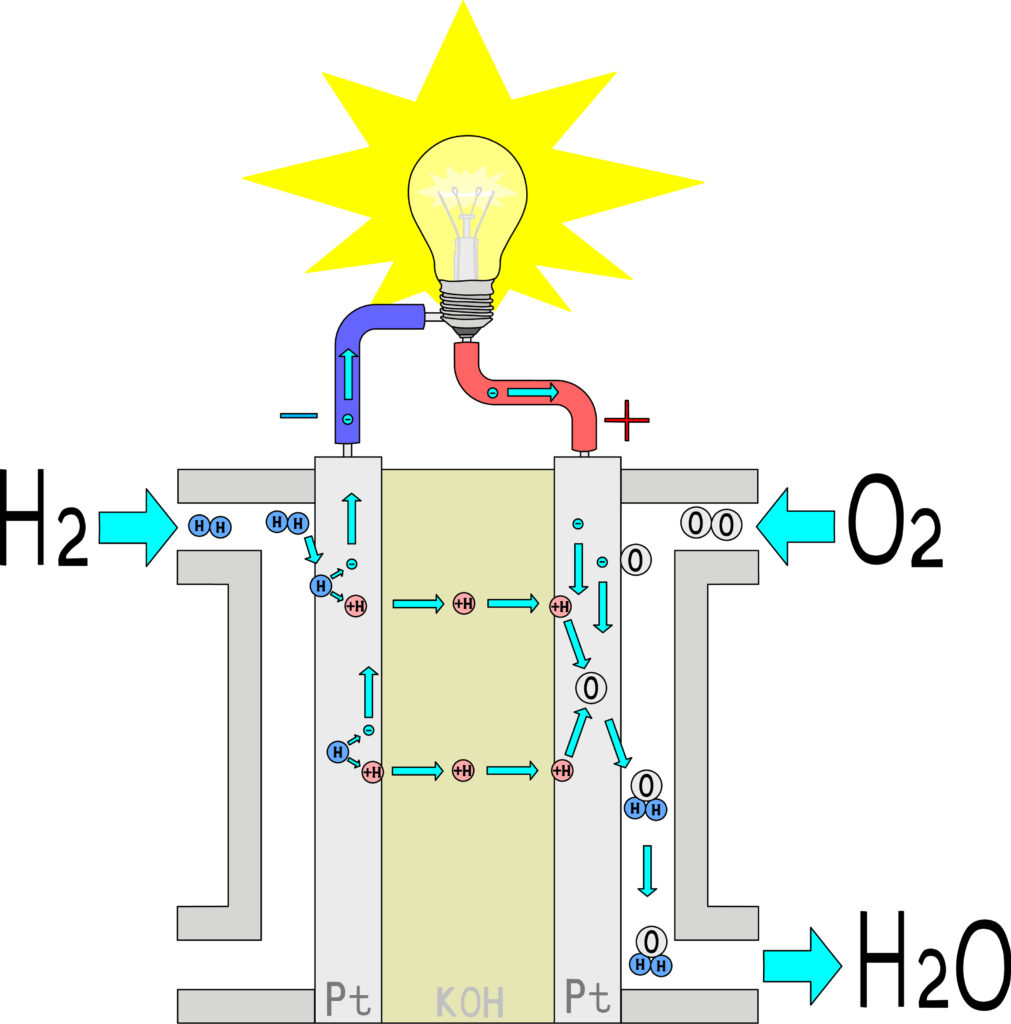
The fuel cell
Hydrogen consumed in a fuel cell, produces electric energy the by driving a turbine just like a power station. Hydrogen can be produced from a variety of domestic resources, such as natural gas, nuclear power, biomass, and renewable power like hydro turbine, solar and wind. Hydrogen can be stored so it can transfer the energy produced from other sources. The fuel cell has become a favored option for all transportation and electricity generation applications.[2]
The hydrogen clean fuel can be produced by a number of diverse methods. The most economical are natural gas reforming (a thermal process), and electrolysis. Other methods are solar-driven and biological processes.
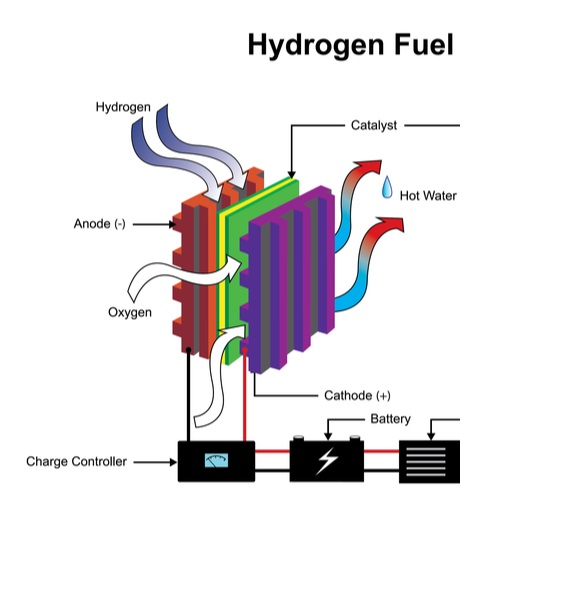
Making Hydrogen
THERMAL PROCESSES
A high temperature processes to produce hydrogen involves a process of steam reacts with a hydrocarbon fuel to produce hydrogen. Many hydrocarbon fuels can be reformed to produce hydrogen, including natural gas, diesel, renewable liquid fuels, gasified coal, or gasified biomass. Today, about 95% of all hydrogen is produced from steam reforming of relatively low-cost natural gas.
Natural gas contains methane (CH4) that can be used to produce hydrogen with thermal processes, such as steam-methane reformation and partial oxidation.
Steam-methane reforming
Methane reacts with steam (7000 to 10000C) under pressure (3–25 bar) in the presence of a catalyst to produce hydrogen, carbon monoxide, and a relatively small amount of carbon dioxide. Heat and pressure must be supplied to the process for the reaction to proceed. A further process of water-gas shift is carried out.
Steam-methane reforming reaction
CH4 + H2O (+ heat) → CO + 3H2
Water-gas shift reaction
The produced carbon monoxide is mixed with more steam and a catalyst to produce carbon dioxide and more hydrogen. Then a final process step called “pressure-swing adsorption,” carbon dioxide and other impurities are removed from the gas stream, leaving essentially pure hydrogen.
Water-gas shift reaction
CO + H2O → CO2 + H2 (+ small amount of heat)
Partial Oxidation
Where natural gas is reacted with air, there is insufficient oxygen to convert the methane and other hydrocarbons completely to carbon dioxide and water. The resultant produces primarily hydrogen and carbon monoxide, nitrogen and a relatively small amount of carbon dioxide and other compounds.
Then in the water-gas shift reaction, the carbon monoxide reacts with water to form carbon dioxide and more hydrogen.
In partial oxidation heat is given off. This method is faster than steam reforming and requires a smaller reactor vessel. However this process initially produces less hydrogen per unit of the input fuel than is obtained by steam reforming of the same fuel.
Partial oxidation of methane reaction
CH4 + ½O2 → CO + 2H2 (+ heat)
Water-gas shift reaction
CO + H2O → CO2 + H2 (+ small amount of heat)
Fuel Cell Electric Vehicle FCEV
Petroleum consumption and emissions from FCEVs are lower than for gasoline-powered internal combustion engine vehicles. Water vapor is said to be the only exhaust product. If you include the production of hydrogen from natural gas, delivery and storage logistics, then atmospheric emissions are halved and petroleum consumption is only 10 percent of petrol driven vehicles.
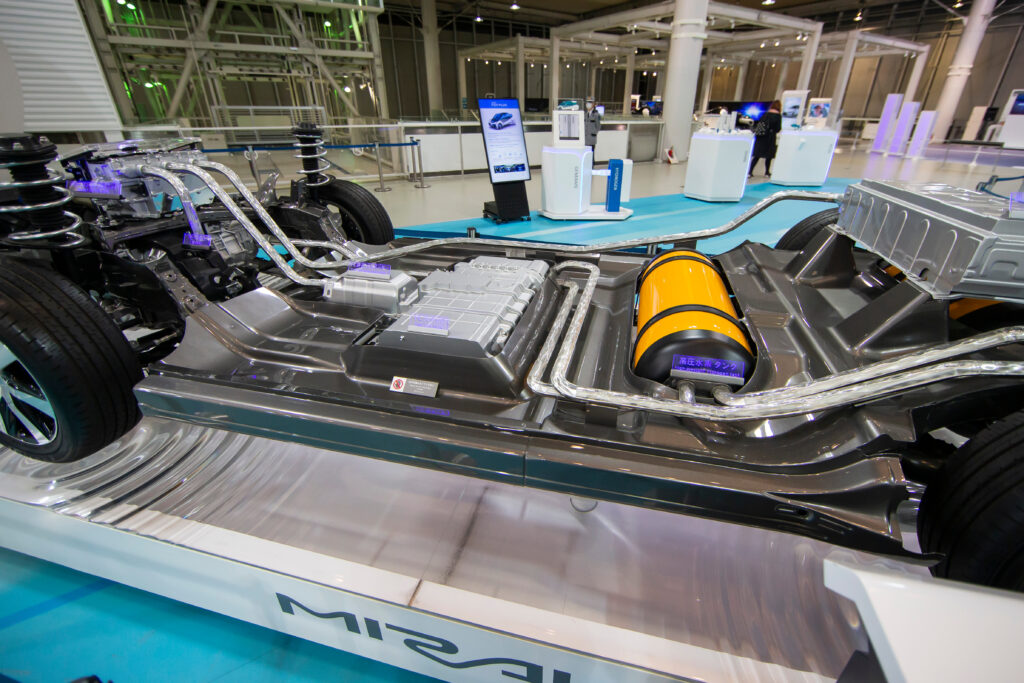
ELECTROLYTIC PROCESSES
Water can be separated into oxygen and hydrogen through a process called electrolysis. Electrolytic processes take place in an ‘electrolyzer’, which functions much like a fuel cell in reverse—instead of using the energy of a hydrogen molecule, like a fuel cell does, an electrolyzer creates hydrogen and oxygen from water molecules.[3]
Hydrogen Production: Electrolysis Electrolysis is a promising option for hydrogen production from renewable resources. Electrolysis is the process of using electricity to split water into hydrogen and oxygen. This reaction takes place in a unit called an electrolyzer which is well-suited for small-scale distributed hydrogen production to large-scale, central production facilities that could be link into a system of other non-greenhouse-gas-emitting forms of electricity production.[4]
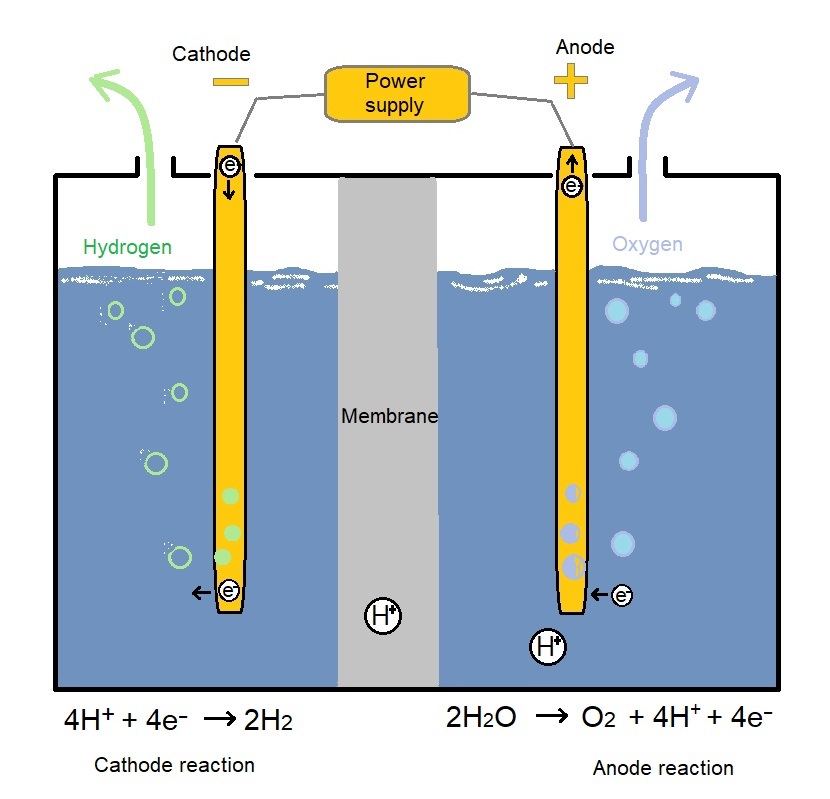
Learn more about electrolytic hydrogen production.
How Does it Work?
Like fuel cells, electrolyzers consist of an anode and a cathode separated by an electrolyte. Different electrolyzers function in slightly different ways, mainly due to the different type of electrolyte material involved.
This Process is being considered because hydrogen produced via electrolysis can result in zero greenhouse gas emissions, depending on the source of the electricity used. The source of the required electricity—including its cost and efficiency, as well as emissions resulting from electricity generation—must be considered when evaluating the benefits and economic viability of hydrogen production via electrolysis.
Amalgamation with renewable electricity generation
Hydrogen production via electrolysis could be working when excess electricity is produced from wind farms or solar installations. Instead of curtailing the electricity as is commonly done, it is possible to use this excess electricity to produce hydrogen through electrolysis. The hydrogen in storage is available to produce electricity when wind or sun is not available.
SOLAR-POWERED Hydrogen Production
Sunlight as the driver for hydrogen production has a few processes, including photo-biological, photo-electro-chemical, and solar-thermo-chemical.
Photo-biological processes use the natural photosynthetic activity of bacteria and green algae to produce hydrogen.
Photo-electro-chemical processes use specialized semiconductors to separate water into hydrogen and oxygen.
Solar-thermo-chemical hydrogen production uses concentrated solar power to drive water splitting reactions often along with other species such as metal oxides.
BIOLOGICAL PROCESSES
Biological processes use microbes such as bacteria and microalgae and can produce hydrogen through biological reactions. In microbial biomass conversion, the microbes break down organic matter like biomass or wastewater to produce hydrogen, while in photo-biological processes the microbes use sunlight as the energy source.
Microbial hydrogen production
Microorganisms have the ability to consume and digest biomass and release hydrogen referred to as microbial biomass conversion processes. Research in biomass conversion could result in commercial-scale systems becoming suitable for local distribution, semi-central, or central hydrogen production scales, depending on the organisms (feedstock) used.[5]

HOW DOES IT WORK?
Microorganisms, such as bacteria, break down organic matter to produce hydrogen. The organic matter can be raw biomass sources like refined sugars, corn stover*, and even wastewater. Because no light is required, these methods are sometimes called “dark fermentation” methods.
(*Stover consists of the leaves, stalks, and cobs of maize plants left in a field after harvest. Such stover makes up about half of the yield of a corn crop and is similar to straw from other cereal grasses.)
Photobiological hydrogen production
Microorganisms —such as green microalgae or cyanobacteria—together with sunlight split water into oxygen and hydrogen ions. Challenges for this pathway include low rates of hydrogen production and the fact that splitting water also produces oxygen, which quickly inhibits the hydrogen production reaction and can be a safety issue when mixed with hydrogen in certain concentrations.
Some photosynthetic microbes use sunlight as the driver to break down organic matter, releasing hydrogen, this is known as photofermentation. The algae and bacteria could be grown in water that cannot be used for drinking or for agriculture, and could potentially even use wastewater. Its challenges include a very low hydrogen production rate and low solar-to-hydrogen efficiency, making it a commercially unviable for hydrogen production. In time photobiological production may provide economical hydrogen production from sunlight with low- to net-zero carbon emissions. [6]
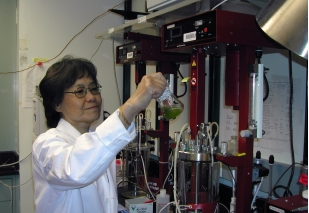
Photobiological hydrogen production from cyanobacteria Anaebena variabilis
The objective of the Samueli Engineering UCLA project is to perform a comprehensive study to simultaneously mitigate carbon dioxide and produce hydrogen. It offers a cheap, efficient, scalable, autonomous, and reliable system for producing hydrogen from microbial consumption of carbon dioxide and absorption of solar light.[7]
How it works

Anabaena variabilis are: filamentous, heterocystous cyanobacteria.
- Feature high hydrogen production capacity in the absence of nitrogen.
- Considered to be good carbon dioxide consumer.
- Approximately 5 mm in diameter and 100 mm long.
- Their genome has been sequenced.
Photobioreactor Description and Operation
The UCLA Engineering project has designed, built, and now are operating a fully instrumented photobioreactor. The following measurements are systematically performed:[8]
Environment | In the liquid phase- | In the gas phase |
Incident light intensity gas in-flow rate | -Temperature – pH, dissolved O2 – nitrates-, ammonia- | out-flow rate, pressure, -gas composition (O2, H2, CO2, and N2 ) |
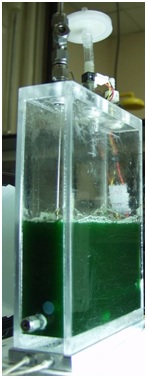
The photobioreactor is operated using two stages. Switching from Stage 1 to Stage 2 takes place when the nitrate concentrations in the liquid phase vanishes.
Stage 1: Carbon Dioxide Consumption and Bacterial Growth
- presence of nitrates and nitrogen.
- sparging with 95% air and 5% CO2 at 170 mL/min.
- irradiance: 65-75 umol/m2/s.
Stage 2: Hydrogen Production
- absence of nitrates and nitrogen.
- sparging with pure Argon at 45 mL/min.
- irradiance: 150 umol/m2/.
Results
- The growth phase lasted 110 hours.
- The H2 production phase lasted more than a week.
- The light to hydrogen energy conversion efficiency reached 0.5%.
- The light to biomass energy conversion efficiency was 4.7%.
Solar Thermochemical Processes
Thermochemical water splitting uses high energy chemical reactions to produce hydrogen and oxygen from water. This is a long-term technology pathway, with potentially low or no greenhouse gas emissions.
The temperatures needed to split water molecules into their atomic constituents are 500° to 2,000°C. The heat could applied from either solar installations or the waste heat of nuclear power the reactions.
Reflective solar installations already in operation.
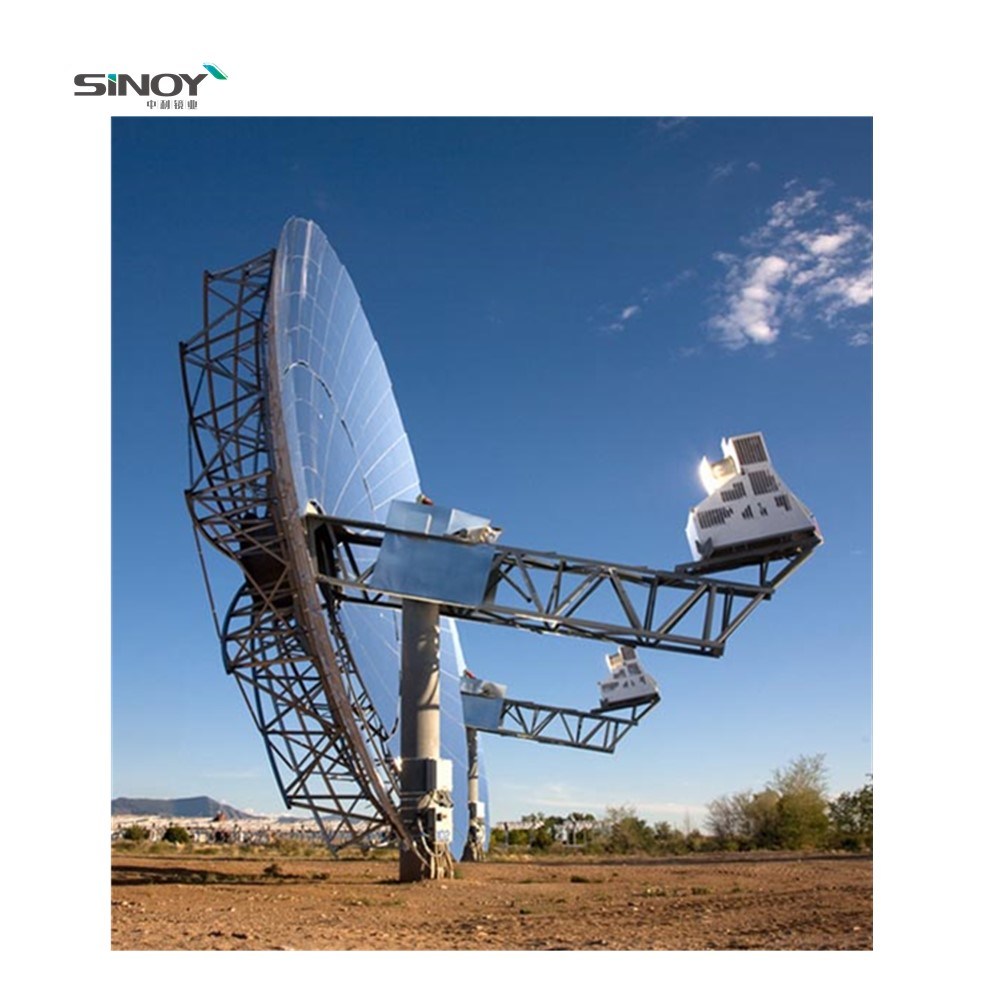
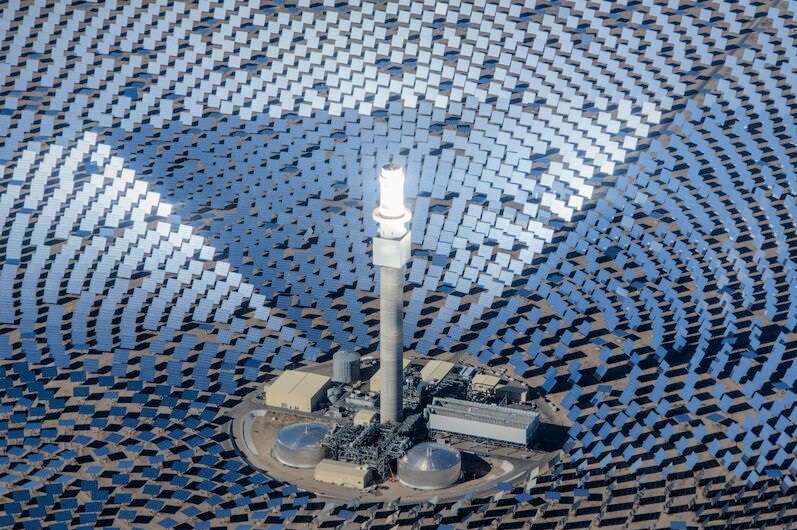
The chemicals used in the process are retained within each cycle, a closed loop that consumes only water and produces hydrogen and oxygen.[9]
At the heart of these water splitting reactors is a thermo- chemical cycle of oxidation and reduction of cerium oxide particles
Reduction: 2Ce(IV)O2 –> Ce(III)2O3 + ½ O2
Oxidation: Ce(III) 2O3 + H2O ® 2Ce(IV)O2 + H2
Net Reaction: H2O –> ½ O2 + H2
Or a multi-step thermo-chemical cycle using copper chloride
Dissociation: 2Cu2OCl2 –> 2CuCl + ½ O2
Hydrolysis: 2CuCl2 + H2O –> 2 Cu2OCl2 + 2HCl
Electrolysis: 2CuCl + 2HCl + (electric input) –> 2CuCl2 + H2
Net Reaction: H2O –> O2 + H2
Thermochemical and Hybrid Water Splitting Technologies
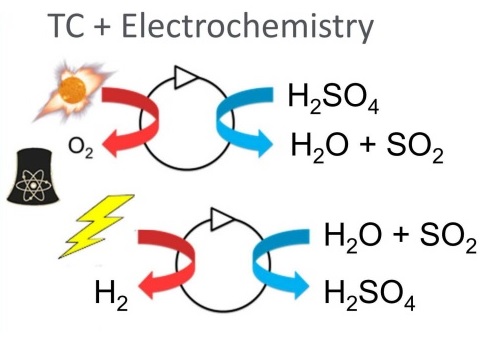
H2SO4 –> H2O + SO2 + ½O2 (Thermochemical; 800-900oC)
SO2 + 2H2O –> H2SO4 + H2 (electrochemical; 80-120oC)
Net Reaction: H2O –> H2 + ½O2 [10]
Photo – Electro – Chemical Processes
Photo-Electro-Chemical Water Splitting: Hydrogen Production
Using photo-electro-chemical (PEC) materials in specialized semiconductors the energy in light causes the molecules in water to break their covalent bonds and separate into hydrogen and oxygen.
This is a long-term approach with the aim of eliminating greenhouse gas emissions.
The semiconductor materials used in the PEC process are similar to those used in photovoltaic solar electricity generation, but for PEC applications the semiconductor is immersed in a water-based electrolyte, where sunlight energizes the water-splitting process. The National Renewable Energy Laboratory provides a demonstration. NREL Photoelectrolysis

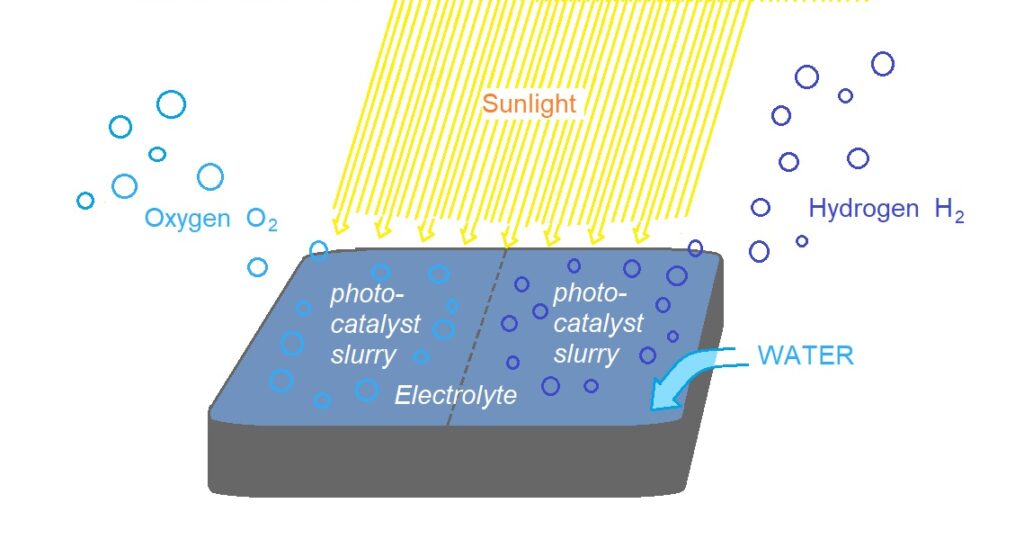
So far panel style reactors have been trialed, owing to the similarities with established photovoltaic panel technologies.[11]
PEC reactor design schemes
Why Is This Pathway Being Considered?
PEC water splitting PEC reactor design schemes achieve high conversion efficiency at low operating temperatures using cost-effective thin-film and/or particle semiconductor materials.
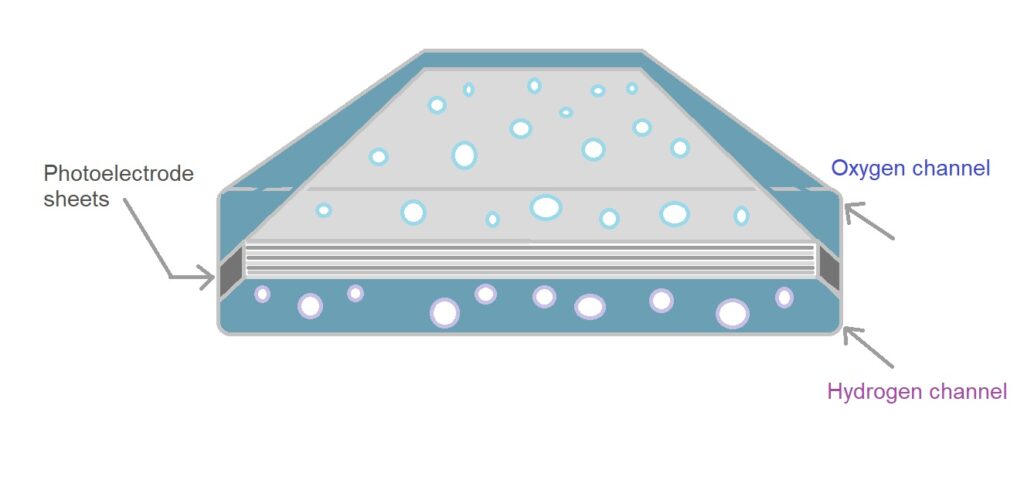
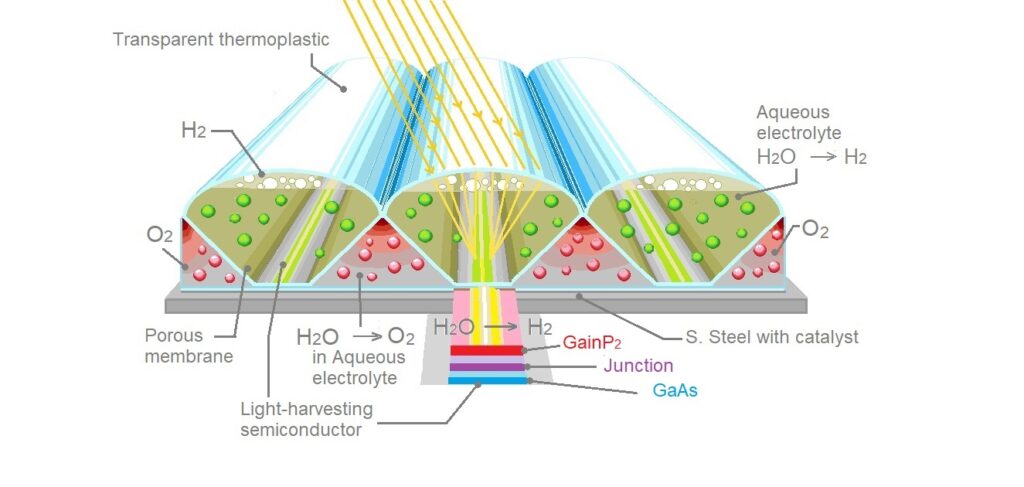
Gallium indium phosphide (GaInP) is a semiconductor. As an electrode its surface could be responsible for the observed corrosion inhibition. Thus, the p-GaInP2 should last much longer when working in pH1 NH4NO3[12]Gallium arsenide (GaAs) is a III-V direct band gap semiconductor with a zinc blende crystal structure.[13]
Particulate Photocatalyst Water-Splitting Panel for Large-Scale Solar Hydrogen Generation.
T. Takewaki holds patents related to this project , published: January 17, 2018. A demonstration of a potentially inexpensive sunlight-powered watersplitting reactor using a fixed Al-doped SrTiO3 photocatalyst. The panel filled with only a 1-mm-deep layer of water capable of rapid release of product gas bubbles without forced convection. A flat panel reactor with 1 m2 of light accepting area could achieved a solar-to-hydrogen energy conversion efficiency of 0.4% by water splitting under natural sunlight irradiation.[14]
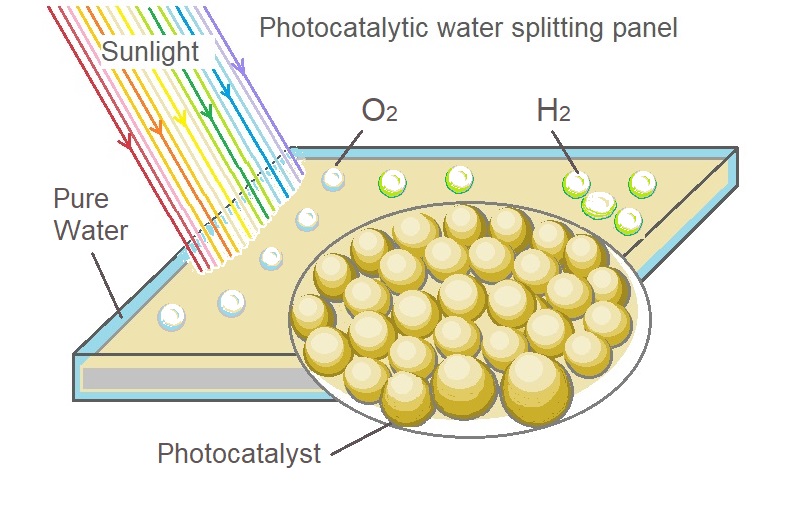
Research Focuses on Overcoming Challenges
Improvements in efficiency, durability, and cost are still needed for market viability. Design parameters receiving attention include,
- Enhanced sunlight absorption and better surface catalysis.
- Durability and lifetime are being improved with more rugged materials and protective surface coatings.
Hydrogen Fuel Cell
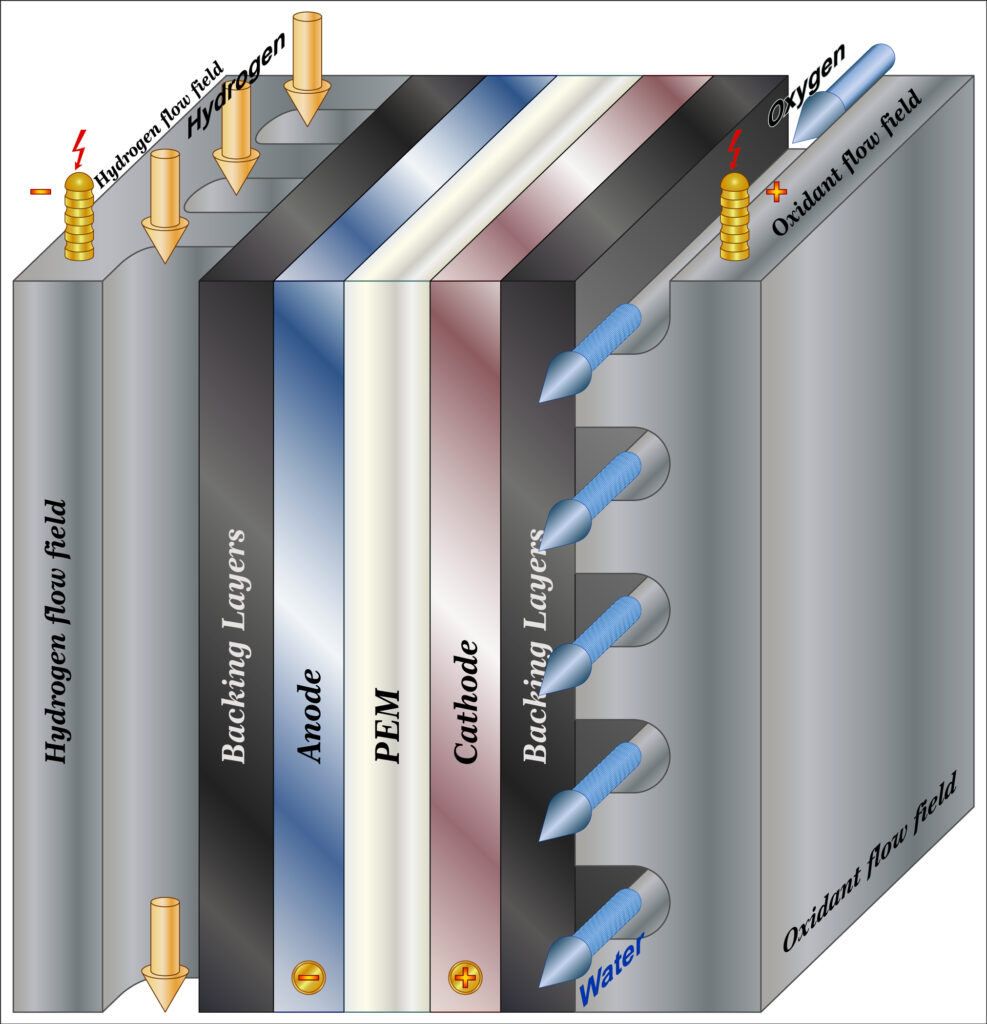
Inside a fuel cell
A fuel cell works much like an electric battery, converting chemical energy into electrical energy using the movement of charged hydrogen ions across an electrolyte membrane to generate current. There they recombine with oxygen to produce water – a fuel cell’s only emission, alongside hot air.
Although less efficient than electric batteries, today’s fuel cells compare favourably with internal combustion engine technology, which converts fuel into kinetic energy at roughly 25 per cent efficiency. A fuel cell, by contrast, can mix hydrogen with air to produce electricity at up to 60 per cent efficiency.
FCEVs also present relatively low barriers to entry in terms of societal changes, as they operate and perform similarly to conventional vehicles, refuelling at stations in minutes and driving for 500 to 600 kilometres on a single tank, all with no harmful emissions.
Making hydrogen
The hydrogen fuel itself can be produced with ever-increasing cost-effectiveness through electrolysis, by splitting water into its constituent hydrogen and oxygen atoms. This generates two useful gases and, when powered by green energy, makes hydrogen production a carbon-neutral enterprise.
At present, however, just 2 per cent of the 600 billion cubic meters of hydrogen manufactured each year around the world is produced by water electrolysis, while 98 per cent is produced from natural gas, with carbon dioxide as a by-product.
More than 90 per cent of this hydrogen is used as a building block for fertilisers or is consumed within the oil, refining and wider petrochemicals industry.[15]
The development of the hydrogen economy, therefore, relies on government investment in the initial phases. Investment in both hydrogen production and distribution infrastructure is needed, alongside the renewable power projects required to supply carbon-neutral energy.
The Toyota Mirai, the world’s most popular FCEV (pictured earlier), was launched in the UAE back in October 2017, when the country’s first hydrogen station was opened by a partnership of local Toyota distributor Al-Futtaim Motors, Abu Dhabi’s Masdar City and France’s Air Liquide.
The hydrogen economy is taking off in Japan, where 100 refuelling stations have already been established, and the government aims to have 800,000 FCEVs on the road by 2030, alongside a 90 per cent reduction in the cost of hydrogen by 2050.
Electric competition
Recent funding into the research and development of electric vehicle (EV) technology has vastly outstripped that of water electrolysis and fuel cell technology.
This situation has been driven by the rapid development of more efficient and cost-effective electric battery technology, lowering the potential costs of electrified transport systems.
EV and hybrid vehicles have an additional edge in terms of overall energy efficiency. Batteries now lose only about 17 per cent of the initial input of electrical energy through inefficiencies when charging and discharging, while the cycle of using electrical energy to split water into its constituent atoms and recombining hydrogen with air inside a fuel cell wastes more than 50 per cent.
Installing EV charging points at key locations, such as at facility car parks, is also simpler and promises governments more immediate and obvious returns than the more complex task of establishing hydrogen distribution infrastructure.
The upshot of this is that, to date, while EV and plug-in hybrid vehicle sales number in the millions, the most popular model of FCEV, the Toyota Mirai, has sold just 5,000 units.
Transport niche
FCEV technology could be especially useful for commercial applications where bulkier vehicles need to travel long distances, carry heavy loads and refuel with minimal downtime.
Trials in hydrogen have been conducted on everything from public buses and forklifts to trains, planes and boats. For such applications, especially with the larger craft, electric batteries would need to be problematically large. Hydrogen could also be used to replace some of the compressed natural gas used in domestic applications, with minimal changes to existing infrastructure.
A study by Swansea University in the UK found that up to 30 per cent of domestic gas could be safely replaced with hydrogen, thereby reducing carbon emissions by 18 per cent, with no changes to existing infrastructure.
In Europe, the ‘EUTurbines’ group of manufacturers has pledged to make their gas turbines run on up to 20 per cent hydrogen gas by 2020, and develop turbine technology to allow all manufactured units to run, or be retrofitted to run, on 100 per cent hydrogen gas, and be carbon-neutral, by 2030.
Rising efficiencies
Globally, the cost of hydrogen is already coming down, partly in line with the fall in the cost of renewable energy, but also due to improvements in water electrolysis and hydrogen fuel cell technology.
The Paris-based International Energy Agency expects the cost of producing hydrogen to fall by a further 30 per cent by 2030, but the rapid reduction in the cost of recent photovoltaic solar energy projects in the Middle East could mean the local cost of commercially producing hydrogen will fall even faster. As investment in hydrogen infrastructure grows and net costs continue to fall, the hydrogen economy could prove to be an indispensable tool in the transition away from hydrocarbons.
[15] Ref: No 15 https://www.power-technology.com/comment/standing-at-the-precipice-of-the-hydrogen-economy/
[14] Ref No 14: https://www.cell.com/joule/pdf/S2542-4351(17)30224-6.pdf
[12] Ref no 12 : https://www.sciencedirect.com/science/article/abs/pii/S0360319912016291
[13] Ref no 13: https://en.wikipedia.org/wiki/Gallium_arsenide
[11] Ref no 11: https://www.energy.gov/eere/fuelcells/hydrogen-production-photoelectrochemical-water-splitting
[10] Ref 10: HydroGEN overview https://www.osti.gov/servlets/purl/1567808
[9] Ref 9:https://www.energy.gov/eere/fuelcells/hydrogen-production-thermochemical-water-splitting
[8] Ref 8: https://www.seas.ucla.edu/~pilon/Bioreactor.html
[7] Ref 7: https://www.seas.ucla.edu/~pilon/Bioreactor.html
[6] Ref 6: https://www.energy.gov/eere/fuelcells/hydrogen-production-photobiological
[5] Ref 5: https://www.energy.gov/eere/fuelcells/hydrogen-production-microbial-biomass-conversion
[4] Ref 4: https://www.energy.gov/eere/fuelcells/hydrogen-production-electrolysis
[3] Ref 3: https://www.energy.gov/eere/fuelcells/fuel-cells
[2 Ref 2: https://www.energy.gov/eere/fuelcells/hydrogen-fuel-basics
[1] Ref 1: Bethe, Hans A. (April 1950). “The Hydrogen Bomb”. Bulletin of the Atomic Scientists. 6 (4): 99–104, 125–. Bibcode:1950BuAtS…6d..99B.
Hydrogen further reading
Mauritus Oil Spill
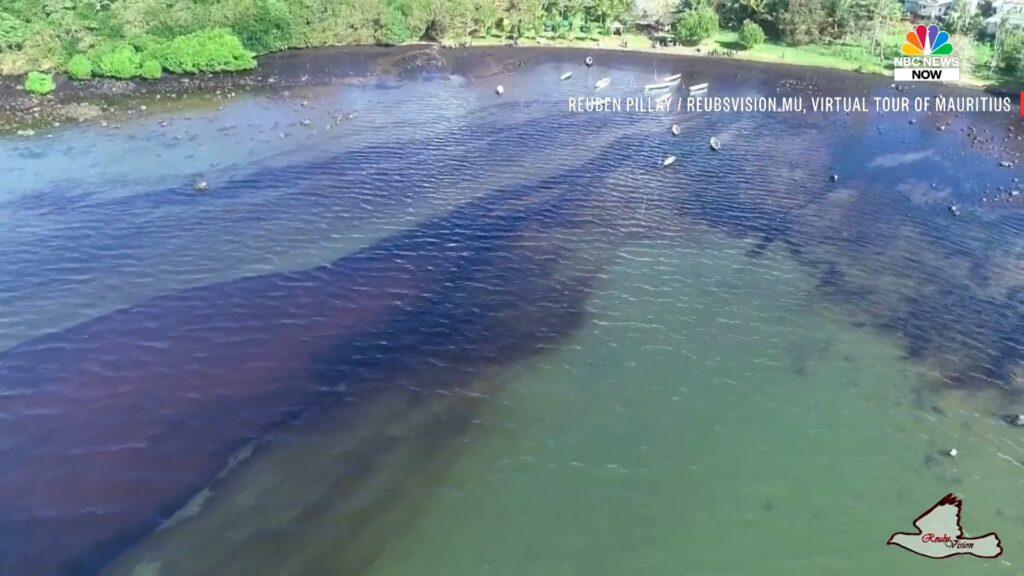
The volunteers have ignored a government order to leave the clean-up operation to local officials, potentially risking a fine or other punishment. NGOs asked volunteers on Tuesday not to risk their health cleaning up the oil on the coast but to concentrate on boom-making instead.
High winds and waves are pounding the Japanese bulk carrier, which is showing signs of breaking up and dumping its remaining cargo into the waters surrounding the postcard-perfect island off the east coast of Africa.

Nearly 2,000 metric tons of oil, diesel and petroleum lubricants could inundate the lagoon if the Wakashio breaks apart, and experts believe it’s a matter of hours.
“The situation is very critical. Cracks have expanded over the course of the day,” said Dr. Vassen Kauppaymuthoo, the island’s premier oceanographer.
“The situation’s about to get 10 times worse. It’ll be a major catastrophe,” he said.
The oil is traveling up the coast, Kauppaymuthoo told NBC News, which could lead to huge stretches of lagoon being affected.
“It’ll take decades to rehabilitate the lagoon, and it’ll never be as it was before the spill. We have thousand-year-old coral here, protected species in our waters,” he added.1
Ref no 1: nbc news
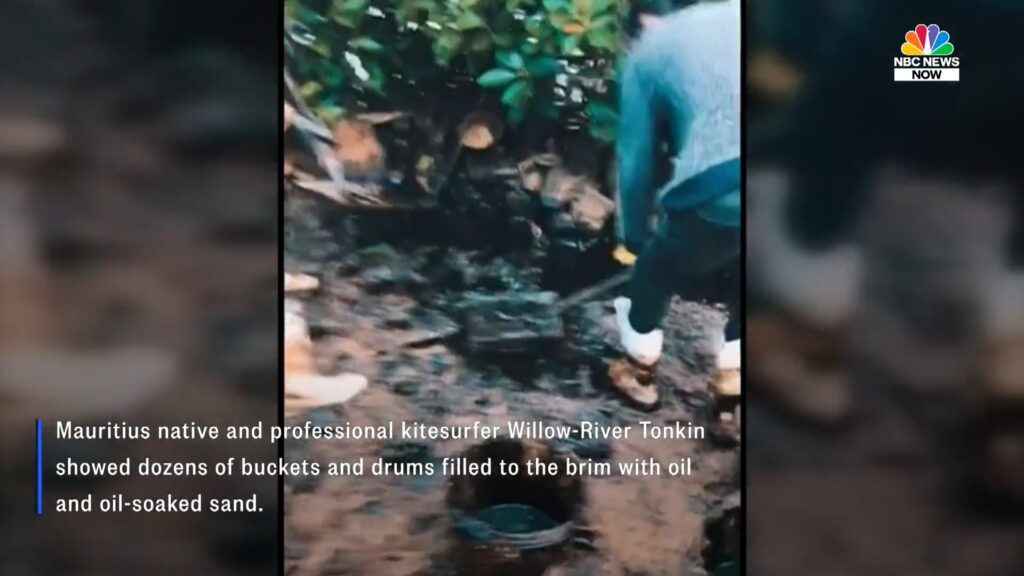
Hydrogen Production: Natural Gas Reforming
Link: Energy.gov. Hydrogen-natural-gas-reforming
Fuel production
Z‐Scheme Photocatalytic Systems for Solar Water Splitting
Boon‐Junn Ng Lutfi Kurnianditia Putri Xin Ying Kong Yee Wen Teh Pooria Pasbakhsh Siang‐Piao Chai
Hitherto, numerous attempts are made to imitate the natural photosynthesis of plants by converting solar energy into chemical fuels which resembles the “Z‐scheme” process.
Link: onlinelibrary.wiley.com
Related Report:
Stored hydrogen
Link: https://www.hydrogenics.com/technology-resources/hydrogen-technology/fuel-cells/
Stored hydrogen can also be used to run heavy duty mobility and everyday fuel cell vehicles. By recombining hydrogen and oxygen, a flow of electrons is created that result in electricity that can be used to run electric engines.
Hydrogen + Oxygen = Electricity + Water Vapor
Pic: pictures>science energy>hydrogen> Hydrogen and oxygen fuel cell , by Fouad A. Saad
reactions: Cathode: O2 + 4H+ + 4e– → 2H2O Anode: 2H2 → 4H+ + 4e– Overall: 2H2 + O2 → 2H2O
A fuel cell is a device that converts chemical potential energy (energy stored in molecular bonds) into electrical energy. A PEM (Proton Exchange Membrane) cell uses hydrogen gas (H2) and oxygen gas (O2) as fuel. The products of the reaction in the cell are water, electricity, and heat. This is a big improvement over internal combustion engines, coal burning power plants, and nuclear power plants, all of which produce harmful by-products.
Since O2 is readily available in the atmosphere, we only need to supply the fuel cell with H2 which can come from an electrolysis process (see Alkaline electrolysis or PEM electrolysis).
The four basic elements of a PEM Fuel Cell:
The anode, the negative post of the fuel cell, has several jobs. It conducts the electrons that are freed from the hydrogen molecules so that they can be used in an external circuit. It has channels etched into it that disperse the hydrogen gas equally over the surface of the catalyst.
The cathode, the positive post of the fuel cell, has channels etched into it that distribute the oxygen to the surface of the catalyst. It also conducts the electrons back from the external circuit to the catalyst, where they can recombine with the hydrogen ions and oxygen to form water.
The electrolyte is the proton exchange membrane. This specially treated material, which looks something like ordinary kitchen plastic wrap, only conducts positively charged ions. The membrane blocks electrons. For a PEMFC, the membrane must be hydrated in order to function and remain stable.
The catalyst is a special material that facilitates the reaction of oxygen and hydrogen. It is usually made of platinum nanoparticles very thinly coated onto carbon paper or cloth. The catalyst is rough and porous so that the maximum surface area of the platinum can be exposed to the hydrogen or oxygen. The platinum-coated side of the catalyst faces the PEM.
New Power Process graphic by Cummins.com
Advantages of the technology:
- By converting chemical potential energy directly into electrical energy, fuel cells avoid the “thermal bottleneck” (a consequence of the 2nd law of thermodynamics) and are thus inherently more efficient than combustion engines, which must first convert chemical potential energy into heat, and then mechanical work.
- Direct emissions from a fuel cell vehicle are just water and a little heat. This is a huge improvement over the internal combustion engine’s litany of greenhouse gases.
- Fuel cells have no moving parts. They are thus much more reliable than traditional engines.
Electrolyzer Generates Green Hydrogen from Wind Power
Link: https://fuelcellsworks.com/news/electrolyzer-generates-green-hydrogen-from-wind-power/
Key Step Toward Cleaner, More Efficient Mass-Production of Hydrogen From Water
Development of cost-efficient electrocatalyst for hydrogen production
Link: https://phys.org/news/2020-10-cost-efficient-electrocatalyst-hydrogen-production.html
New insight brings sustainable hydrogen one step closer
Link: https://phys.org/news/2020-10-insight-sustainable-hydrogen-closer.html
Microwaving plastic waste can generate clean hydrogen
Link: https://www.newscientist.com/article/2256822-microwaving-plastic-waste-can-generate-clean-hydrogen/
Scientists Build Tiny Microbial Factories That Produce Hydrogen by Photosynthesis
Eco-Friendly Fuel Cells Powered by Instant Hydrogen Production
Link: https://scitechdaily.com/eco-friendly-fuel-cells-powered-by-instant-hydrogen-production/
Zero-emission fuel: Tackling storage issues of hydrogen
Link : https://blog.novomof.com/blog/zero-emission-fuel-tackling-storage-issues-of-hydrogen
Solar, hydrogen and fuel cells combined › H2-international
Link: https://www.h2-international.com/2020/05/15/solar-hydrogen-and-fuel-cells-combined/
Comments are closed.