Wetlands emit methane by a natural process. The microbes in the water saturated soil produce the methane. Recently a scientist from GNS Te Pû Ao announced “What we have found is an extremely tough methane-consuming organism,“ that could be used to reduce methane emissions from landfills and geothermal power stations. [14] I thought ‘there lies the solution’, but is it that simple?
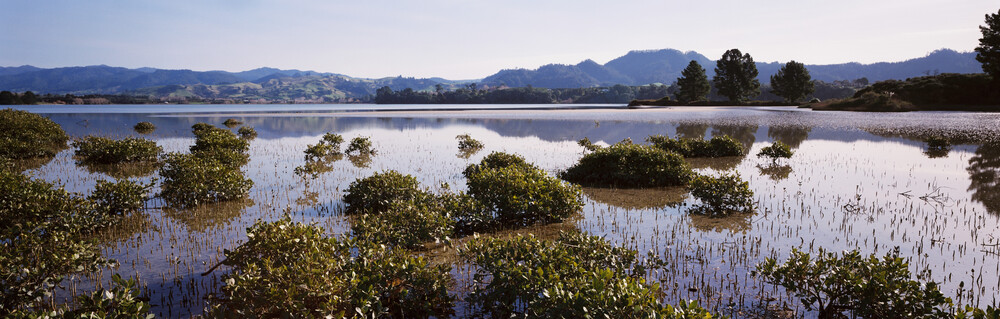
Wetland emissions are the greatest contributor to the total airborne methane. About 1/3 of all methane from natural and human activities (anthropogenic) come out of the world’s wetlands. Unfortunately those emissions are getting bigger and can be attributed to higher temperatures and the melting of frozen tundra. It is referred to as ‘climate feedback’. [6]
Methane Matters
A significant number of anthropogenic processes like landfill, agriculture, and fossil fuel, apart from natural systems like wetlands emit methane. It is the second most important greenhouse gas we produce, contributing approximately 167 million of metric tons of methane to the atmosphere each year. [5]
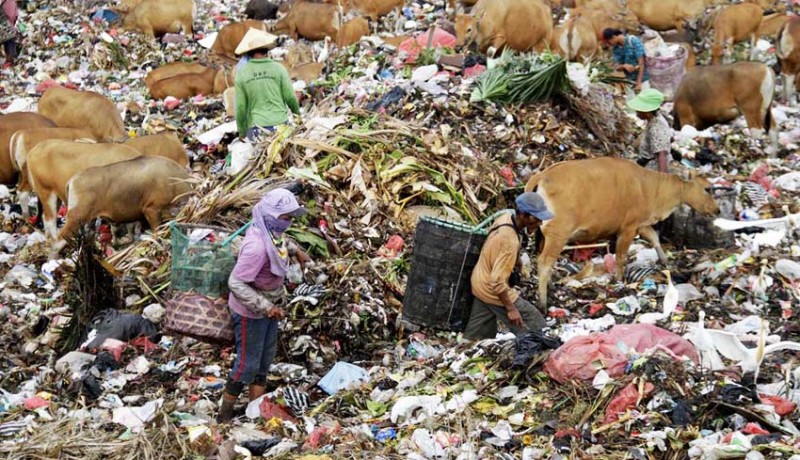
Methane has an impact 25 times greater than carbon dioxide on global warming. From the industrial age methane supplied 25% of the climate-warming effect of greenhouse gases.
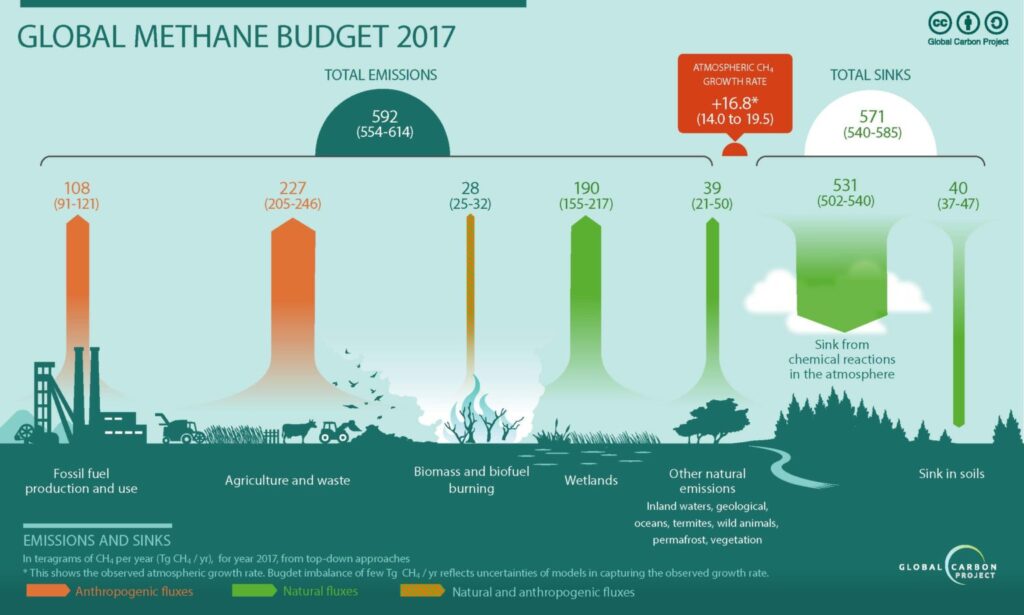
Fortunately methane has a much shorter lifetime in the atmosphere than CO2. If we can dramatically reduce our emissions methane will decline relatively quickly. [1]
Wetland Regions
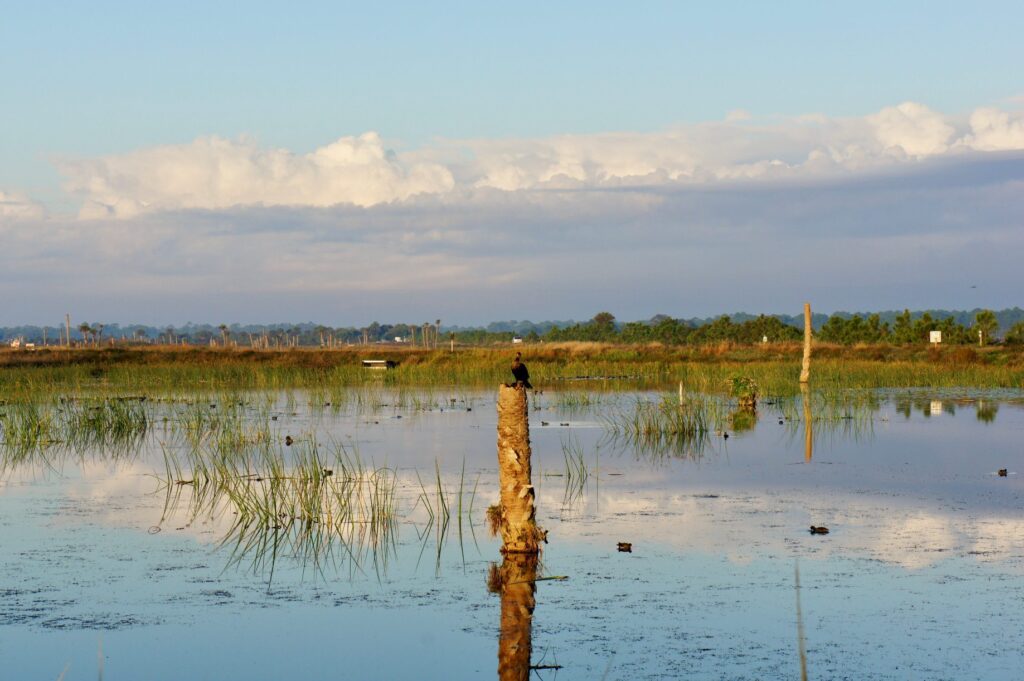
A wetland is an ecosystem that manages water with a complex variety of plants, soil and aquatic life forms that serves to capture and cleanse water. Human settlement continues to expand into wetlands, draining them to construct a built environment and agriculture.
Cutting drains through wetlands disconnects the natural flow and retention of water. Towns historically built on the banks of rivers. They are now cities with a civil engineering issue with storm water run-off. A solution to wetland draining is to rebuild these wetlands in another area more convenient to humans. These ‘constructed wetlands’ are often built along original creek beds. They could be located on storm water lines or rebuild an area no longer used for agriculture. They regulate water run-off and mitigate flood damage.[2]
Swamp, Bog or Marsh?
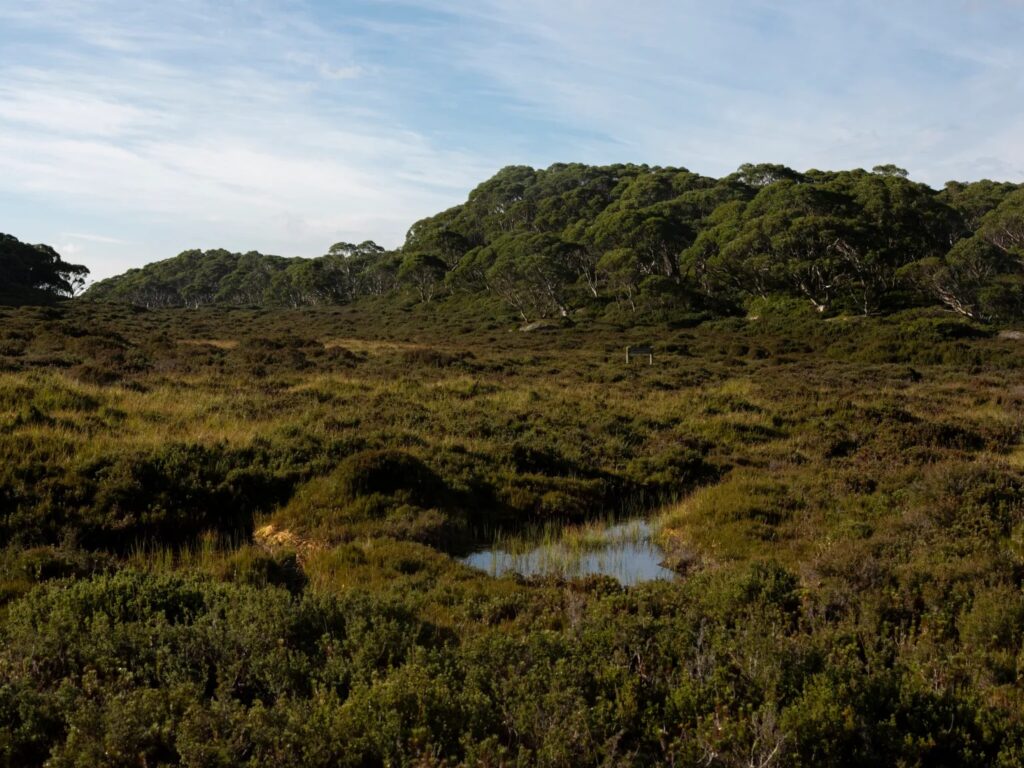
Wetlands are often classified by landscape position, vegetation, and hydrologic regime. Wetland classes include marshes, swamps, bogs, fens, peatlands, muskegs, pocosins and prairie pothole. [22] Characteristics of wetland classes can assist to inform on magnitude of methane emissions. However, wetland classes have displayed high variability in methane emissions spatially and temporally.
How Wetlands emit methane.
Wetlands have a poor oxygen environment and emit methane. In that wet and relatively warm environment the microbes consume oxygen more rapidly than it can absorb in from the atmosphere. The microbes in the soil are forced to metabolize under anaerobic conditions, wetlands are the ideal conditions for fermentation as well as methane production.
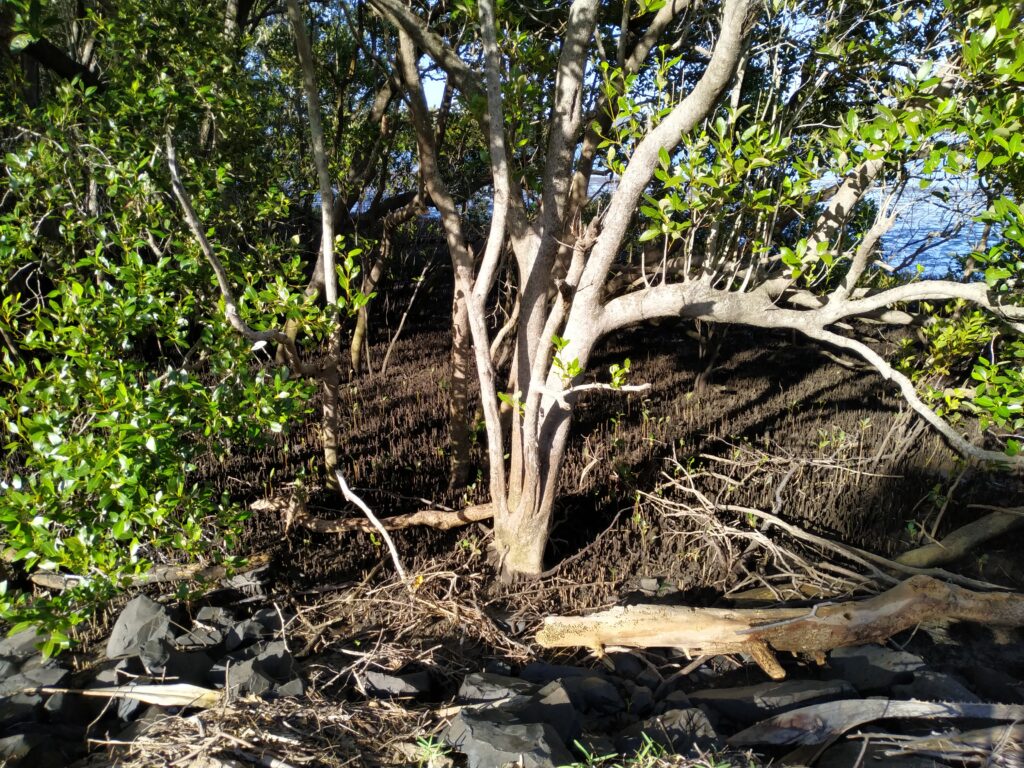
During anaerobic respiration, other terminal electron acceptors are used instead of oxygen. Nitrate may be converted to NH4, sulfate or molecular sulfur to H2S, CO2 to CH4 methane, ferric ion to ferrous ion, and fumarate to succinate.[3]
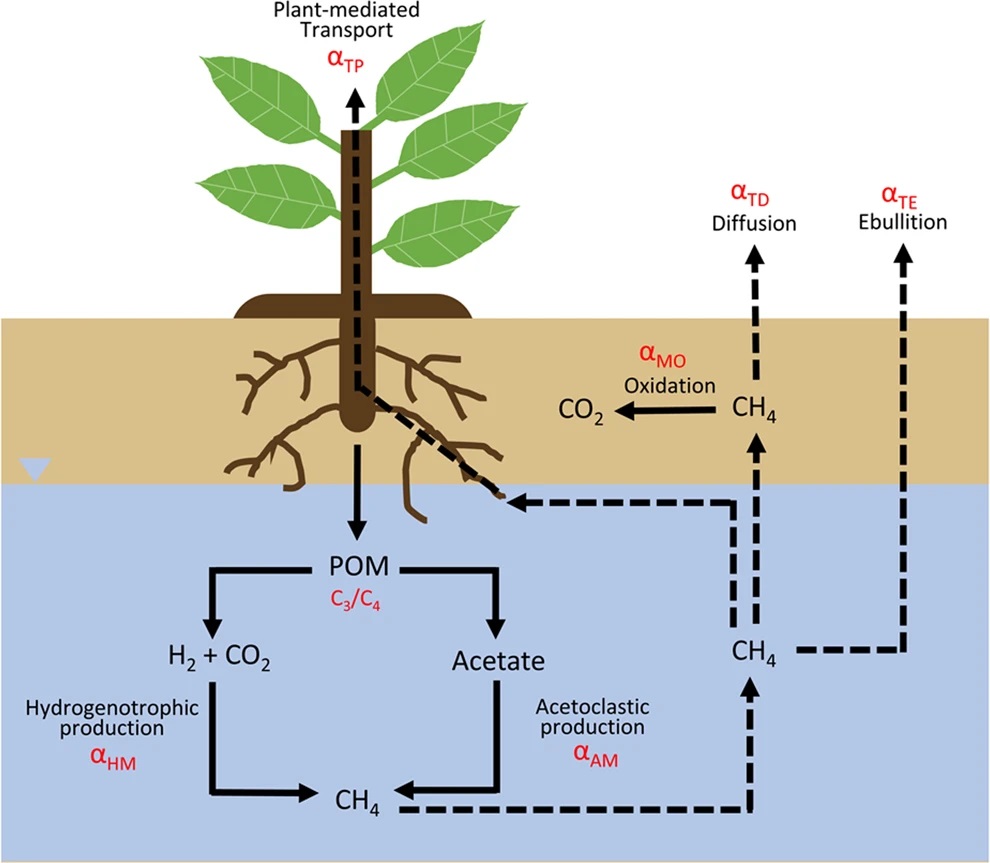
Fermentation is a process used by certain kinds of microorganisms to break down essential nutrients. In a process called acetoclastic methanogenesis, microorganisms from the classification domain archaea produce methane by fermenting acetate and H2-CO2 into methane and carbon dioxide.[4]
H3C-COOH → CH4 + CO2
Depending on the wetland and type of archaea, hydrogenotrophic methanogenesis, another process that yields methane, can also occur. This process occurs as a result of archaea oxidizing hydrogen with carbon dioxide to yield methane and water.[10]
4H2 + CO2 → CH4 + 2H2O
Diffusion
The diffusion through the profile refers to the movement of methane up through soil and bodies of water to reach the atmosphere. The importance of diffusion as a pathway varies per wetland based on the type of soil and vegetation [12]. For example, in peatlands, the mass amount of dead, but not decaying, organic matter results in relatively slow diffusion of methane through the soil [11]. Additionally, because methane can travel more quickly through soil than water, diffusion plays a much bigger role in wetlands with drier, more loosely compacted soil.
The methane is diffused into the atmosphere through the tissue of plants seen as a release of gas bubbles. The hydrologic stability of wetland soils, as well as the transport efficiency through plants, can affect how much and how often methane is released from the soil. A hydrodynamic study aims to find out if a given flow is stable or unstable, and if so, how these instabilities will cause turbulence [8].

Karla Jarecke and researchers from several universities have been studying wetlands’ impact on methane gas.
“Understanding the conditions under which methane is produced and released in wetlands could lead to solutions to reduce methane emissions,” says Jarecke. Wetlands are difficult places to work, so Jarecke and her colleagues made “mesocosms” of wetlands, outdoor chambers where methane emissions could more easily be measured. At their wetland mesocosm sites in Lincoln, Nebraska looked at two common plants; swamp milkweed and northern water plantain.
Comparisons of the methane emissions of the two plant species showed little difference in the field. Soil saturation proved a greater variable in methane emissions.
Ebullition
Ebullition refers to the spasmodic release of bubbles of methane into the air. These bubbles occur as a result of methane building up over time in the soil, forming pockets of gas. As these pockets of trapped methane grow in size, the level of the soil will slowly rise up as well. This phenomenon continues until so much pressure builds up that the bubble bursts, pushing the methane up through the soil so quickly that it does not have time to be consumed by the methanotrophic organisms (discussed below) in the soil. With this release of gas, the level of soil then falls once more. [13]
Environmental factors
Temperature
It’s a lot warmer in the tropics, so you get a lot of biological activity and more production of methane than from the high latitudes where it’s really cold. We’ve estimated annual emissions totaling over 110 million tons from tropical wetlands versus about 10 million tons from the high latitudes. [1] Those emissions are natural, so they will continue, as long as we don’t drain the wetlands, which do happen.
Water table
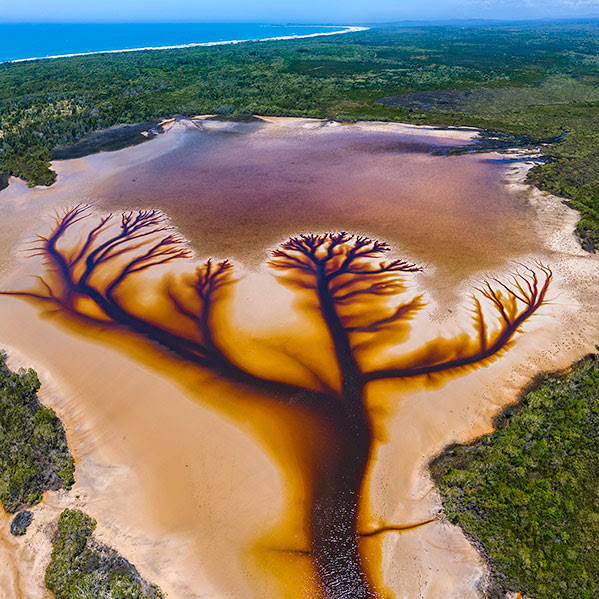
Further research is needed to understand how varying soil saturation affects methane emissions. This information could be valuable for designing wetland topography that creates hydrologic conditions for increased carbon storage and reduced methane emissions.[9]
Soils
Researchers studied the effects of hydrology — or the saturation of the soil. “While the controls of hydrology and plant species on methane emissions are individually well-studied, the two are rarely studied together,” says Jarecke
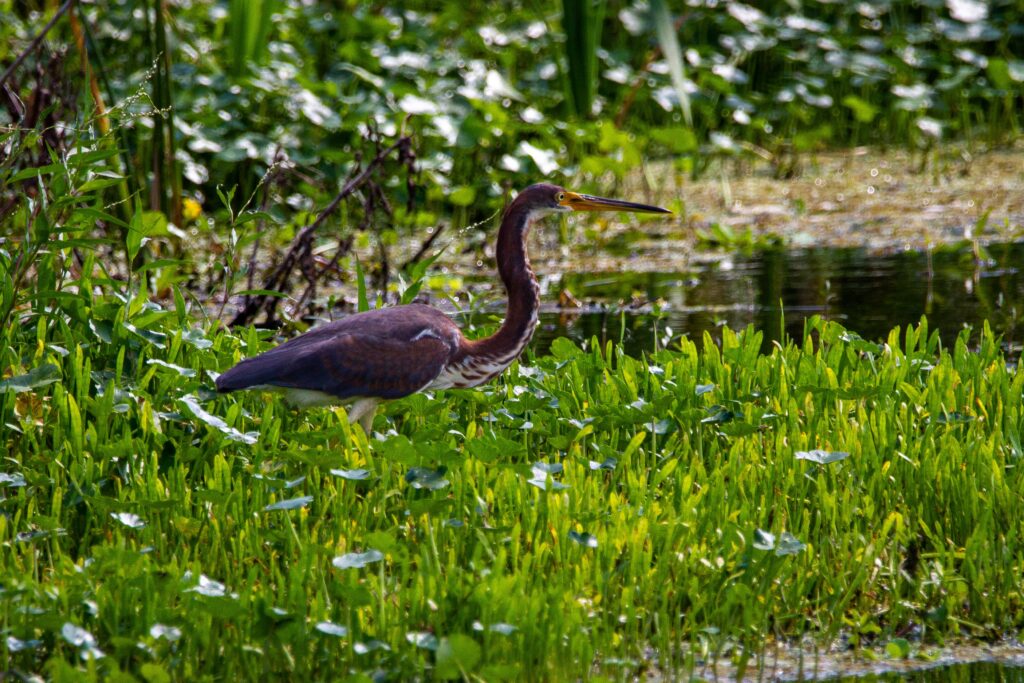
Finding plant species that reduce microbial methane production could be a key to better wetland management. For example, plants that deliver oxygen to the rooting zone can suppress microbial methane production. . “Methane emissions likely change as restored wetlands mature. Organic matter from root systems, decaying plants and other materials will build up. This helps restore hydrologic stability,” said Jarecke. Other research indicates that it can take just a few years to restore hydrologic aspects of a restored wetland. However, biogeochemical and biodiversity aspects can take decades or longer to recover.” [9]
Human development of wetlands
Studying large areas like wetlands can prove impossible. So, Karla Jarecke and her colleagues made “mesocosms” of wetlands — manageable, outdoor chambers where methane emissions could more easily be measured. Mesocosms are structural research areas that bridge the gap between lab studies and large field studies. [9 ]
Human activities such as marsh draining for agriculture are increasingly eating away at saltwater and freshwater wetlands that cover only 1% of Earth’s surface but store more than 20% of all carbon. [19]
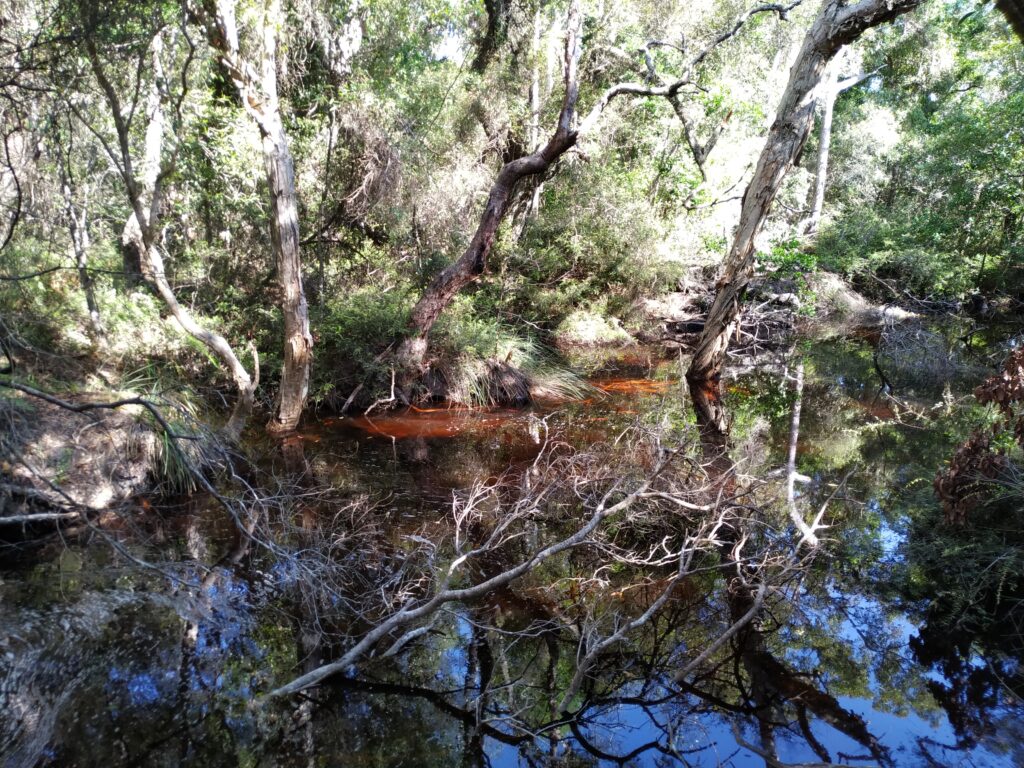
New studies provided by Flinders University show valuable insights into removing toxins from polluted waterways and improving filtration at urban wetlands. One study found a wetland plant capable of reducing Per- and Polyfluoroalkyl Substances (PFAS) in soil and water, and another looked for better urban wetland water flow management during summer. [20]
Methanotroph
Methanotrophs (sometimes called methanophiles,not to be confused with Methanogen, ) are prokaryotes (a single-celled organism that lacks a nucleus) that metabolize methane as their source of carbon and chemical energy.
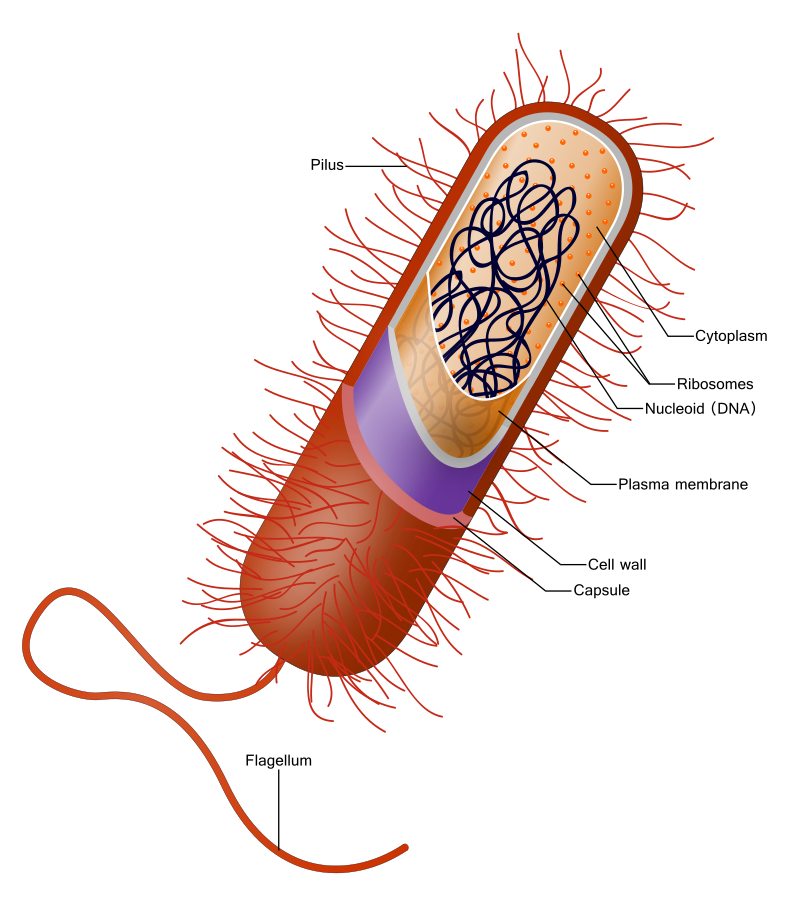
They are Bacteria (formerly Eubacteria) or Archaea (formerly Archaebacteria), can grow aerobically or anaerobically (with or without oxygen), and require single-carbon compounds (like methane) to survive. Methanotrophs are common around environments where methane is produced, and use the gas as their only source of energy. Their habitats include wetlands, soils, marshes, rice paddies, landfills, aquatic systems (lakes, oceans, streams) and alike. They are of special interest to researchers studying global warming, as they play a significant role in the global methane budget [6], by reducing the amount of methane emitted to the atmosphere. [16][17]
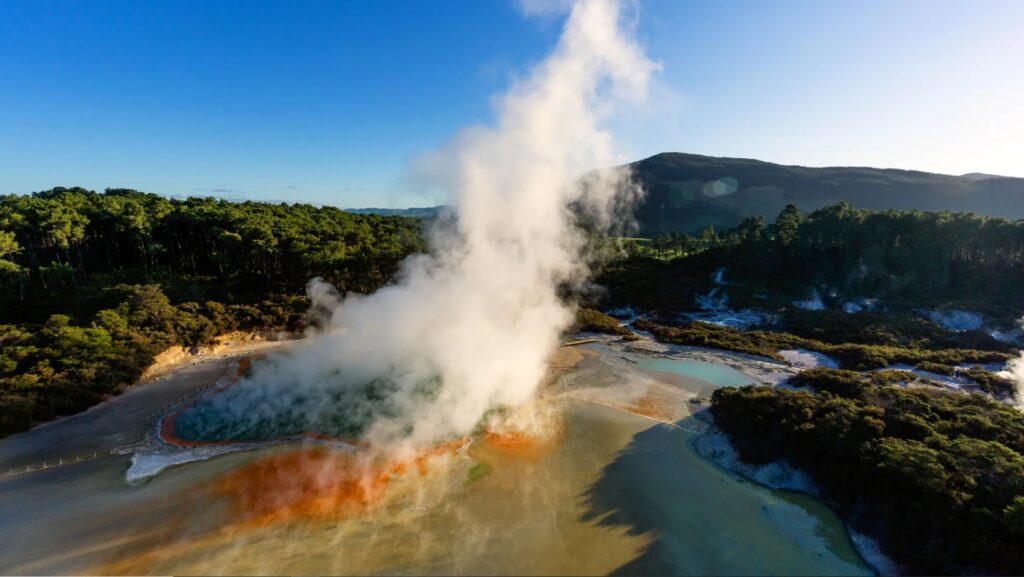
In 2007 a methane-consuming microorganism that lives in geothermal areas in Rotorua has attracted international attention for its ability to live in extremely acidic conditions. Discovered by researchers at GNS Science, the bacterium could one day be used to reduce methane gas emissions from landfills. It could also help to cut methane emissions from geothermal power stations. The hardy bacterium is part of the methanotrophs group, but this one is able to live in hotter and much more acidic conditions than its relatives. [14]
Microbiologist at GNS Science, Matthew Stott, identified its international significance.
“We knew methane was being produced geothermally at Hell’s Gate and we were puzzled as to why it wasn’t reaching the surface … What we have found is an extremely tough methane-consuming organism that is new to science. It grows happily under extremely acidic conditions in the lab,” said Dr Stott.
Scientists have always suspected that a proportion of this methane was being consumed by bacteria living in these environments and without them, the amount of methane entering the atmosphere would be much greater.
“Ultimately, it may be possible to implant this organism, or a similar one, in landfills and cut methane emissions into the atmosphere.”
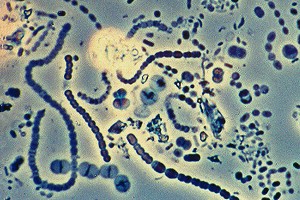
Fellow microbiologist at GNS Science, Peter Dunfield, who isolated the bacterium, has tentatively named it Methylokorus infernorum, which describes its food source, and the ‘hellish’ location of its discovery, and also a description of a structure within its cell that resembles a Koru. [14]
GNS Science worked with colleagues at the University of Hawaii to sequence the genome of the bacterium. They found its genetic makeup was different to all known methanotrophic organisms.
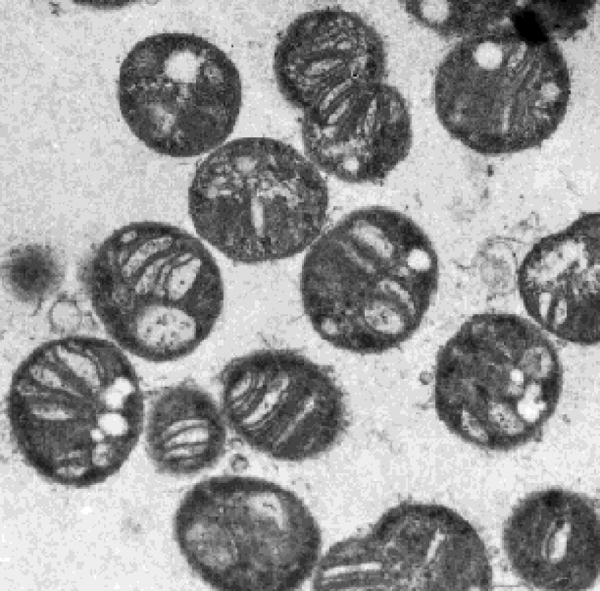
Later renamed Methylococcus capsulatus it can be used to produce animal feed from natural gas. [14] “Methylococcus capsulatus can be used to make edible vaccines, so-called oral vaccines*>Incomestible bacteria The bacterium Methylococcus capsulatus (M. capsulatus) differs from many other bacteria in that it has little of the substances that can be toxic to humans, among others. That makes it a suitable vaccine candidate. [23]
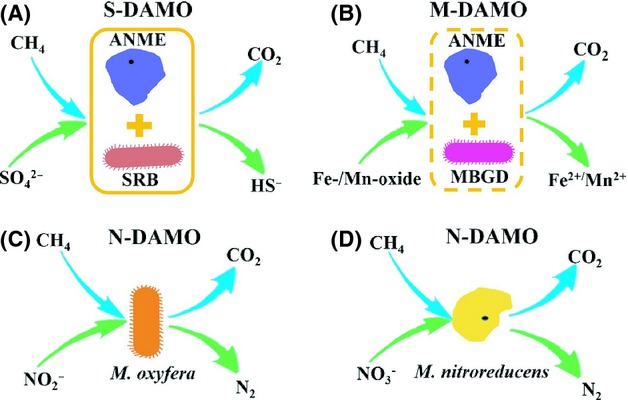
(A) sulphate-dependent anaerobic methane oxidation (S-DAMO);
(B) metal ion (Mn4+ and Fe3+) dependent anaerobic methane oxidation (M-DAMO);
(C, D) nitrate/nitrite-dependent anaerobic methane oxidation (N-DAMO).
ANME, an anaerobic methanotrophic archaea;
SRB, sulphate-reducing bacteria;
M. Oxyfera, Candidatus Methylomirabilis oxyfera;
M. Nitroreducens, Candidatus Meyhanoperedens nitroreducens; MBGD, marine benthic group D.]
References
1. Global Methane Emissions Soaring, But How Much Was Due to Wetlands? Q&A with Berkeley Lab scientist William Riley with Priyanka Runwal August 13, 2020
2. Unexpected culprit: Wetlands as source of methane. June 19, 2019 American Society of Agronomy
3. Bacterial Metabolism and Genetics. Patrick R. Murray PhD, F(AAM), F(IDSA), in Medical Microbiology, 2021
4. Katz B. (2011). “Microbial processes and natural gas accumulations”. The Open Geology Journal. 5 (1): 75–83. Bibcode:2011OGJ…..5…75J. doi:10.2174/1874262901105010075.
5. Plant Species and Hydrology as Controls on Constructed Wetland Methane Fluxes. Cain Silvey, Karla M. Jarecke, Kristine Hopfensperger, Terrance D. Loecke, Amy J. Burgin. Soil Science Society of America Journal, 2019; 0 (0): 0 DOI: 10.2136/sssaj2018.11.0421
6. “Global Methane Budget”. Global Carbon Project. Retrieved 4 December 2018. Note; The methane feedbacks were not fully assessed by the Intergovernmental Panel on Climate Change Fifth Assessment in their report.
7. Emerging role of wetland methane emissions in driving 21st century climate change Zhen Zhang https://orcid.org/0000-0003-0899-1139 [email protected], Niklaus E. Zimmermann, Andrea Stenke, Xin Li, Elke L. Hodson, Gaofeng Zhu, Chunlin Huang, and Benjamin Poulter.
Edited by Wolfgang Lucht, Potsdam Institute of Climate Impact Research, Potsdam, Germany, and accepted by Editorial Board Member Hans J. Schellnhuber July 26, 2017
114 (36) 9647-9652 https://doi.org/10.1073/pnas.1618765114
8. Drazin (2002), Introduction to hydrodynamic stability
- Cain Silvey, Kristine Hopfensperger, Terrance D. Loecke, Amy J. Burgin and Karla M. Jarecke College of Forestry, Oregon State University. Plant Species and Hydrology as Controls on Constructed Wetland Methane Fluxes. Soil Science Society of America Journal, 2019; 0 (0): 0 DOI: 10.2136/sssaj2018.11.0421
- Conrad, Rolf (1999). “Contribution of hydrogen to methane production and control of hydrogen concentrations in methanogenic soils and sediments”. FEMS Microbiology Ecology. 28 (3): 193–202. doi:10.1016/s0168-6496(98)00086-5.
- Couwenberg, John. Greifswald University. “Methane emissions from peat soils.” http://www.imcg.net/media/download_gallery/climate/couwenberg_2009b.pdf
- Tang J., Zhuang Q., White, J.R., Shannon, R.D. (2008). “Assessing the role of different wetland methane emission pathways with a biogeochemistry model”. AGU Fall Meeting Abstracts. 2008: B33B–0424. Bibcode:2008AGUFM.B33B0424T
- Glaser, P.H., J.P. Chanton, P. Morin, D.O. Rosenberry, D.I. Siegel, O. Ruud, L.I. Chasar, A.S. Reeve. 2004. “Surface deformations as indicators of deep ebullition fluxes in a large northern peatland.”
- Methane eating microbe holds promise for greenhouse gas 22/11/2007 GNS Science
- Ettwig KF, Butler MK, Le Paslier D, Pelletier E, Mangenot S, Kuypers MM, et al. (March 2010). “Nitrite-driven anaerobic methane oxidation by oxygenic bacteria”
- Oremland RS, Culbertson CW (1992). “Importance of methane-oxidizing bacteria in the methane budget as revealed by the use of a specific inhibitor”. Nature. 356 (6368): 421–423. Bibcode:1992Natur.356..421O. doi:10.1038/356421a0. S2CID 4234351.
- Holmes AJ, Roslev P, McDonald IR, Iversen N, Henriksen K, Murrell JC (August 1999). “Characterization of methanotrophic bacterial populations in soils showing atmospheric methane uptake”. Applied and Environmental Microbiology. 65 (8): 3312–8. Bibcode:1999ApEnM..65.3312H. doi:10.1128/AEM.65.8.3312-3318.1999
- https://en.wikipedia.org/wiki/NC10_phylum
- Land-Building Marsh Plants are Champions of Carbon Capture | Nicholas School of the Environment (duke.edu)
- New ways to improve urban wetlands: Aquatic plant to remove PFAS, better filtration systems — ScienceDaily
- Bubier, Jill L. and Moore, Tim R. (1994). “An ecological perspective on methane emissions from northern wetlands.” Trends in Ecology & Evolution. 9 (12): 460-464. doi:10.1016/0169-5347(94)90309-3.
- Tangen Brian A., Finocchiaro Raymond G., Gleason Robert A. (2015). “Effects of land use on greenhouse gas fluxes and soil properties of wetland catchments in the Prairie Pothole Region of North America”. Science of the Total Environment. 533: 391–409. Bibcode:2015ScTEn.533..391T. doi:10.1016/j.scitotenv.2015.06.148. PMID 26172606
- Develops edible vaccine from bacterium (forskning.no)